This article has been reviewed according to Science X's editorial process and policies. Editors have highlighted the following attributes while ensuring the content's credibility:
fact-checked
trusted source
proofread
Type VI secretion systems: Weapons of bacterial destruction
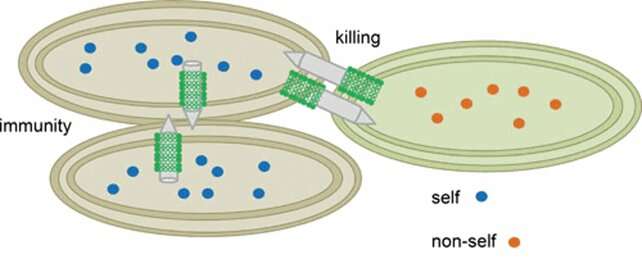
Bacteria can be quite aggressive. Armed with an impressive array of mechanical and biochemical weapons, they don't mess around when it comes to combating their foes. Notable among these armaments is the Type VI Secretion System (T6SS), a membrane-embedded nanomachine found in many gram-negative bacteria. The needle-like system helps bacteria antagonize prokaryotic and eukaryotic cells by injecting them with harmful proteins (effectors), such as pore-forming toxins and nucleases.
T6SSs also promote bacterial survival in other ways, including facilitating acquisition of resources (e.g., metals). As researchers learn about these microbial weapons, they are exploring how they may be used to generate vaccines, synthesize novel antimicrobials and more.
Stab and shoot: T6SS structure and mechanism
The T6SS is one of nine known bacterial secretion systems that transport proteins from inside of a bacterial cell to the external environment, or into another cell. Each secretion system varies slightly in its structure, activity and purpose—the primary function of the T6SS is to destroy other bacteria, but it also modulates bacterial interactions with eukaryotic cells.
Genes encoding T6SSs are found in over 25% of gram-negative bacterial species. The system consists of a contractile sheathed needle that spans the inner and outer membrane of the bacterial cell. The time and place of T6SS activation depends on various environmental cues, including quorum sensing, pH and temperature changes, among others. When the T6SS is deployed, the needle shoots out to stab neighboring cells and deliver deadly effectors into microbial adversaries. This allows bacteria to kill their competitors and free up space and resources for their own use.
In general, hauling around a bunch of toxic compounds is risky. Bacteria harboring one or more T6SS—Yersinia pestis, the causative agent of plague, has up to six—must be able to protect themselves from the effectors they carry. Often, protection comes in the form of immunity proteins. These proteins bind to an effector in the cell and render it inactive. Immunity proteins can also shield bacteria from attack by other cells: if a bacterium has the immunity protein(s) that match an attacker's effector(s), it is protected. In this way, bacteria are prevented from killing their kin, which will have the requisite immunity proteins, while eliminating "non-self" cells. There are also immunity protein-independent forms of self-protection and defense, such as the activation of stress responses.
T6SS functions in prokaryotic communities
T6SS-mediated warfare helps determine which microbes survive in intensely competitive environments, such as the gut. Here, thousands of bacteria vie for a limited number of spatial and nutritional niches; those with T6SSs may have a leg up on their competitors. For example, Bacteroides fragilis, an abundant member of the intestinal microbiota, uses its T6SS to antagonize other human gut Bacteroidales (the order to which B. fragilis and most gram-negative gut bacteria belong).
Within this vein, T6SSs promote colonization resistance against pathogenic bacteria and help the interlopers to overcome it. A recent study showed that the mouse intestinal pathogen, Citrobacter rodentium (a model for human pathogenic Escherichia coli) uses a T6SS to colonize the mouse gut by targeting commensal Enterobacteriaceae. Resident E. coli species, in turn, use T6SSs to combat invasion by C. rodentium. Human pathogens, like Salmonella enterica serovar Typhimurium and Vibrio cholerae, also use their T6SSs to kill resident microbial species and weasel their way into the community.
The role of the T6SS in colonization resistance is not limited to animal hosts—it also applies in the context of plant-associated microbiota and pathogens. Moreover, T6SSs serve other functions besides modulating bacterial mortal combat. For example, bacteria can secrete proteins from the T6SS that fetch metal ions (e.g., zinc and iron, which are important in numerous cellular processes) from their environment and return them to the cell, thus enabling resource acquisition and survival. In addition, T6SS-mediated lysis of prey cells releases DNA that can be taken up by the attacker, which may facilitate interbacterial transfer of antibiotic resistance genes.
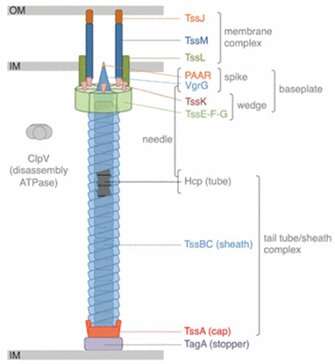
T6SS effectors and eukaryotic cells
Although the T6SS is primarily linked to its antibacterial powers, it can also target eukaryotic cells (e.g., mammalian cells and fungi). In this regard, T6SS-secreted effectors have important implications for pathogen virulence and infection. In fact, when the T6SS was first discovered in V. cholerae in 2006, it was shown to secrete proteins required for bacterial cytotoxicity toward mammalian macrophages. Only later did its antibacterial capabilities come to light.
Since then, various T6SS effectors have been shown to influence host responses to and virulence of bacterial pathogens. For example, Enterohemorrhagic E. coli (EHEC), a foodborne pathogen, secretes a catalase via its T6SS that facilitates its survival in macrophages. The catalase, KatN, reduces macrophage production of reactive oxygen species that damage bacterial cells. Francisella tularensis, an intracellular pathogen and the cause of tuleremia, similarly secretes a T6SS effector that tempers levels of the proinflammatory cytokine, TNF-α, in monocytes in vitro, hinting at its potential immunomodulatory function.
T6SSs also regulate fungal-bacterial relations and, in doing so, may influence the outcome of host-microbe interactions. For instance, Acinetobacter baumanii releases a DNase from its T6SS that targets the nuclei of fungal cells, leading to cell death. Another example: Serratia marcescens, an opportunistic pathogen, releases antifungal effectors that disrupt the membranes and nutrient uptake of fungal cells, including pathogenic Candida species.
Harnessing the T6SS
T6SSs do a lot of work for bacteria, and now scientists are looking at how they can work for humans too. In a 2016 study, researchers showed that fusion of an antigen (i.e., a protein capable of generating an immune response) to the T6SS sheath proteins from E. coli and V. cholerae resulted in T6SS-based nanoparticles that could serve as delivery vehicles for vaccines. Moreover, scientists recently expressed a T6SS in the non-pathogenic bacterium, V. natriegens, that could be turned on/off via an external signal (i.e., the sugar arabinose). The T6SS-based system could be easily manipulated to release a diverse range of effectors and inform development of new antimicrobial treatments.
The T6SS itself may be a viable target when developing novel antibiotics. Such antimicrobials could effectively "disarm" pathogenic bacteria by preventing the release of T6SS-associated virulence factors. The system also has implications in biocontrol (i.e., the use of organisms to control plant pathogens). For example, the T6SS of Pseudomonas putida, a soil bacterium and well-established biocontrol agent, is central to the microbe's ability to kill pathogens. As such, the T6SS mechanism of biocontrol should be considered when selecting and developing agents moving forward.
For now, the above uses for the T6SS remain at the conceptual stage. Still, as is the case for anything in science, there is much to learn about the structure and function of T6SSs in diverse bacterial species. With this understanding will come a firmer grasp on its potential applications.
Provided by American Society for Microbiology